Impact of glycosylation on the effector functions of monoclonal antibodies: a case study of rituximab
Bioanalytics, Biologics, Drug substance, Manufacturing
KEY POINTS
- Glycosylation pattern of monoclonal antibodies may affect nearly every aspect of their pharmacology, starting from pharmacokinetics, and ending with biological activity and therapeutic profile. This is also the case for rituximab, a widely used, chimeric anti-CD20 antibody.
- Many different glycoforms that affect the effector functions and pharmacokinetics of rituximab have been identified. High galactosylation level increases the CDC activity, while high afucosylation correlates with increased ADCC activity. Such changes are likely to boost efficacy, but at the same time be detrimental to safety. Sialylation of rituximab has negligible impact on all parameters.
- Maintenance of the appropriate glycosylation profile is a critical issue during biosimilar drug development. It can be achieved by tweaking the cell culture conditions.
Background
Glycosylation is one of the most widespread post-translational modifications of antibodies, having a critical role in determining their physicochemical properties and functionality1. Addition of glycan moieties takes place in mammalian cell lines such as CHO, which are frequently used to produce therapeutic immunoglobulins. Glycosylation occurs predominantly in Fc fragment and constitutes around 2-3% of the total molecular mass of an antibody2.
There are two types of protein glycosylation: N-linked and O-linked. The first one involves the single-step addition of 14 monosaccharide chain to the -CONH2 group of asparagine (Asn), which later undergoes modification to create the final glycoform. In natural IgGs, glycans are added at Asn297 position within the consensus sequence of Asn-X-Thr/Ser (X can be any amino acid other than proline)2. In contrast to N-linked, O-linked glycosylation entails the sequential addition of monosaccharide to the hydroxyl group present in serine and threonine. Typically, N-acetylgalactosamine is added as the first monosaccharide3.
The influence of glycosylation is determined by the glycan composition as well as their location within the protein structure4. Most common N-glycoforms are displayed in Figure 1 below. These are: G0, G0F, G1, G1F, G2F, G2FS1, G2FS2, M5 and M6, where G stands for galactose, F for fucose, S for sialic acid (N-acetylneuraminic acid in case of rituximab) and M for mannose (see Figure 1)5. Glycosylation is an extremely important parameter influencing the key effector functions of monoclonal antibodies: antibody-dependent cell-mediated cytotoxicity (ADCC), complement-dependent cytotoxicity (CDC) and antibody-dependent cellular phagocytosis (ADCP). In addition, it may influence their pharmacokinetic properties2,3. Examples of associations between various glycosylation patterns and antibody functionality are provided in Table 1.
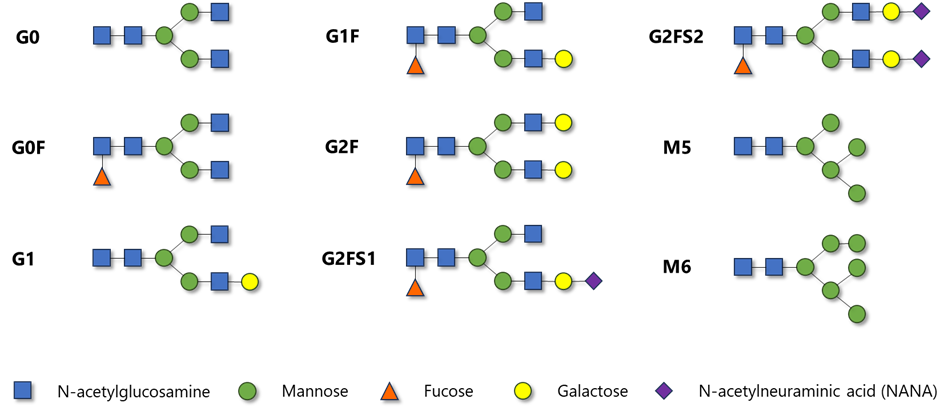
Figure 1 Chemical structure of most common N-glycoforms encountered in monoclonal antibodies.
Due to the tremendous importance of glycosylation profile, monitoring of glycans content is essential during the mAb manufacturing process6. It also has to be taken into consideration during the development of a biosimilar product – glycosylation is regarded as a crucial quality attribute7. Although small differences between the biosimilar and reference may be accepted by regulators, the pharmaceutical company has to prove that their impact on pharmacology is not clinically meaningful8.
Table 1 Potential impacts of the selected glycosylation patterns on pharmacology and clinical properties of monoclonal antibodies2,3,9.
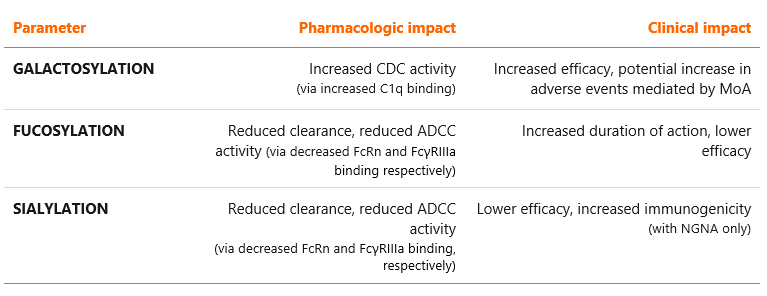
We will demonstrate the above points by looking at the example of rituximab, a chimeric monoclonal antibody commonly used for the treatment of rheumatic and oncologic conditions, and its biosimilars. This antibody works by binding to the CD20 antigen expressed on the surface of B cells, causing their lysis and depletion from the bloodstream10. Rituximab belongs to IgG1 subclass and consists of two heavy chains of 451 amino acids and two light chains of 213 amino acids11.
Case study: rituximab
Major glycan species present in rituximab molecules are G0F, G1F and G2F, followed by low amounts of G0, Man5 (M5), G1, G1F+NANA (G1FS1), G2F+NANA (G2FS1), G2F+2NANA (G2FS2) and other minor constituents (see Figure 2)12,13. As in other IgGs, the N-linked glycans of this antibody reside at the highly conserved asparagine position (Asn297). One of the best analytical methods for analyzing the glycosylation pattern, employed also at Mabion, is HILIC-UHPLC-FLD. Association between the contents of respective glycoforms and pharmacokinetics or pharmacodynamics of rituximab has been extensively studied and described in the scientific literature, as shown in the following sections.
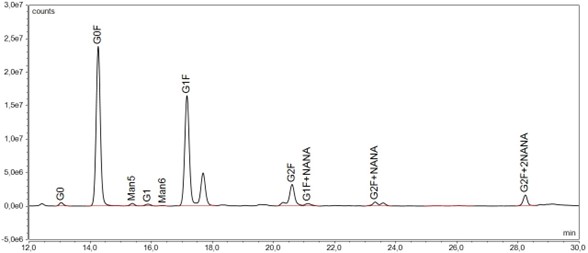
Figure 2 Glycosylation profile of rituximab as assessed by HILIC-UHPLC-FLD.
Galactosylation
Owing to their high diversity, galactose-containing glycans are most often evaluated with the use of total galactosylated species parameter. It has been shown that the removal of terminal galactose from Fc-glycans has no impact on the biologic activity of rituximab mediated by ADCC, but can result in up to 40% reduction of the CDC activity14,15. Terminal Gal residues in Man-α1,6 arm, but less so in Man-α1,3 arm, increase effector functions mostly by potentiating C1q binding and conferring structural stability to the CH2 domain. Experiments have shown that rituximab with added galactose residues (containing only G2 glycoforms) has twice the affinity to C1q protein as antibody that contains only G0 glycoforms16. Data from our internal experiment measuring correlation between galactosylation and CDC activity of rituximab (using preparations of EU-approved MabThera and a candidate biosimilar to rituximab, before and after reducing the content of galactosylated glycoforms), are presented in Table 2. In line with the literature data, the obtained results demonstrate that reduction of galactosylated glycoforms from ~50-60% to ~5-6% leads to a decrease in CDC activity from ~100% to ~60%13.
Table 2 Influence of galactose removal on CDC activity of rituximab. Candidate biosimilar and MabThera samples were subjected to enzymatic digestion with B-GA. Digested, non-digested as well as spiked samples were tested for CDC potency. The effect was tested on three different CD20-positive human cell lines.
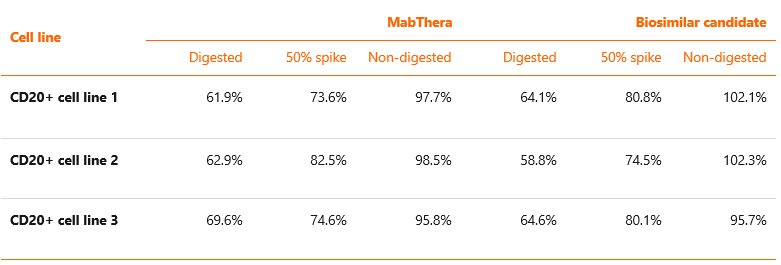
Fucosylation
Fucosylated structures, mostly G0F, G1F and G2F, have the highest average abundance in rituximab molecule of all glycoforms17. Also, in contrast to the endogenous IgGs, rituximab contains substantial levels of high-mannose glycans (0.1% vs. 1%-2%, respectively), a property common to all recombinant antibodies18.
Fucosylation pattern has moderate, but still significant, impact on the pharmacokinetics of mAbs3. It is also a major determinant of their biological activity. Antibodies with a higher level of Man5 (one of the afucosylated species) are more rapidly cleared from blood and redistributed to the liver than those with a more complex glycosylation pattern19. However, large differences in Man5 content are required to exert significant impact on rituximab clearance. For this reason, the total sum of afucosylated species, which can vary within the range of 2.5% – 4.7%13, is thought to have little influence on the overall PK properties of this antibody.
Fucose-containing glycoforms display a strong inverse association with ADCC activity, which is considered to be the most important MoA of rituximab (next to CDC). Afucosylated IgGs have been shown to exhibit an enhanced ADCC activity20. The ADCC-boosting phenomenon can be explained by increased binding of afucosylated mAbs to FcγRIIIa receptor, a protein expressed on the surface of NK cells that interacts with Fc part of antibodies. Studies run internally by Mabion on different rituximab products have fully confirmed this hypothesis – the content of afucosylated species is associated with ADCC potency, with G0 displaying the strongest correlation13,30.
Sialylation
Terminal sialylation of antibodies may affect their antigen binding as well as effector functions. Antibodies with high content of sialylated glycoforms tend to have decreased affinity to FcγRIIIa receptor and in consequence reduced ADCC potency21,22. However, whereas naturally occurring antibodies are sialylated at the level of less than 10%, recombinant antibodies produced in mammalian cell lines usually have only negligible sialylation9. It follows then that slight changes in the amount of sialylated species should have little effect on the biologic activity of rituximab.
Most studies failed to detect any direct impact of sialylation on the PK properties of mAbs23. On the other hand, there is substantial evidence that some sialylated glycoforms increase their immunogenicity. The two most common sialic acids encountered in biopharmaceuticals are N-acetyl-neuraminic acid (Neu5Ac, or NANA) and N-glycolyl-neuraminic acid (Neu5Gc, or NGNA). Humans cannot synthesize Neu5Gc and therefore its high content could elicit strong reaction from the immune system and lead to the emergence of anti-drug antibodies (ADAs) and associated complications such as chronic inflammation24. The synthesized anti-Neu5Gc antibodies might then promote drug clearance, altering the PK profile in an indirect manner3. However, NGNA is present in rituximab only in trace amounts25. In consequence, the content of sialylated glycoforms should have no impact on its PK properties.
Glycosylation patterns
Different patterns of glycosylation may induce conformational changes in mAbs that could have a direct impact on Fc effector functions, including ADCC and CDC26-28. Structural changes may also affect the frequency of ADA formation, increasing or decreasing it depending on the exact nature of these changes. Additionally, asymmetric glycosylation of mAbs could compromise their thermal stability29.
Glycosylation site occupancy
Observations also point to an important role of glycosylation site occupancy in determining the efficacy, safety and immunogenicity of therapeutic antibodies, including rituximab.
The panoply of different glycoforms and glycosylation patterns makes it extremely difficult to predict the overall impact of their changes on mAb’s functionality. In case of rituximab, this uneasy task has been taken up by Mabion’s scientists, who developed and validated a complex mathematical model for predicting the impact of glycosylation on ADCC activity and FcγRIIIa binding. In the paper published in the Journal of Pharmaceutical Sciences, our team reported that the obtained values were in good agreement with the experimental data, the deviations being no higher than 17%30. These results show that in-silico modelling of the glycosylation impact on effector functions of monoclonal antibodies is feasible and could be successfully implemented into the process of biologic drug development. Click here to read our publication.
Glycosylation during the biosimilar development
Glycosylation is considered as one of the most important quality attributes of biosimilars because of its widespread impact on pharmacology of therapeutic proteins. For this reason regulatory agencies all over the world demand the sponsors to present high-level evidence of similarity between biosimilar and reference product with respect to the glycosylation profile31,32. However, the immense number of glycan structures and variability in post-translational modifications, which may shift due to even minor variations of process properties such as cell culture and environmental conditions, make it extremely difficult to develop a biosimilar with a similar glycosylation pattern to the originator’s33. Meeting this objective requires thorough knowledge and high proficiency in developing and optimizing the downstream process.
Fortunately, regulatory agencies tend to accept some dissimilarities in the content of particular glycoforms, provided that no clinically significant impact is anticipated with respect to the efficacy, safety and immunogenicity profile of the product7.
Modulation of glycosylation profile at various phases of manufacturing
During the development of biosimilar drug, the glycosylation issue should be addressed from the very initial stages of mAb production. In fact, upstream processes have the greatest impact on the final glycan composition of biosimilar.
The choice of cell line is utterly critical. It determines the enzyme set (glycosidases and glycosyltransferases) that is involved in the process of glycoform maturation as well as its cell distribution34. CHO, SP2/0, NS0 and Y0 are currently the most commonly utilized cell lines for the development of pharmaceuticals, with notable emphasis on the application of CHO cell line35. CHO is widely known for high similarity and consistency of glycosylation patterns toward human cells. It is also worth noting that in addition to the choice of cell line itself, antibody the glycosylation profile is also affected by other factors such as the cell culture mode and bioreactor type36. Selection of cell culture conditions is another significant element of upstream stage of production with substantial impact on glycosylation profile. Some studies indicate that changes in culture parameters such as decrease in pH lead to increased levels of galactosylation and sialylation, while temperature lowering promotes increased occupancy of glycosylation sites34. Nevertheless, modification of conditions may have different effects depending on the expression system and the produced biomolecule. Furthermore, medium composition and supplementation also make an essential contribution to modulating the glycosylation profile. This approach involves changes in the concentration of sugar substrates, that are the building blocks for glycans, and also using supplementation additives, which alter activity of enzymes, such as cofactors and inhibitors.
The effect of medium composition and supplementation on the glycosylation profile of rituximab molecule was also studied at Mabion. Figure 3 presents the relative sums of galactosylated (GAL), afucosylated (AFU) and sialylated (SIAL) glycoforms of antibody produced by the same cell line cultured with the use of different media (1, 2 and 3) and supplements (A, B and C).
It is notable that different combinations of media and supplements can strongly affect the glycoform group levels. In case of GAL and SIAL, changes in culture composition have induced up to 3-4-fold reduction in relative content with potential implication for antibody CDC activity and clearance, respectively. For afucosylation, the differences in relative glycoform content are not as pronounced as for GAL and SIAL. However, even such minor changes in AFU content may have an important impact on ADCC activity of antibody.
Conceptually, it is also possible to modify the glycosylation profile of mAb through downstream processes optimization. Various types of affinity chromatography based on lectins or FcγRIIIA receptor are described for separation of specific carbohydrate moieties or afucosylated glycoforms, respectively35. Nevertheless, due to the structural and manufacturing complexity of the ligands for antibody molecule binding, these techniques are not cost-effective for large, industrial scale processes.
Therefore, the upstream process of biopharmaceutics production is the most crucial factor for modulation of antibody glycan composition. For biosimilar development, it is vital not only to have knowledge of strategies to control the glycosylation profile but also to know and target specific ranges for glycoform content of the reference drug, in order to ensure adequate safety and efficacy of biosimilar molecule. In this regard, screening of reference molecules to create the Quality Target Product Profile (QTPP) should be executed as early as possible, for any biosimilar development project.
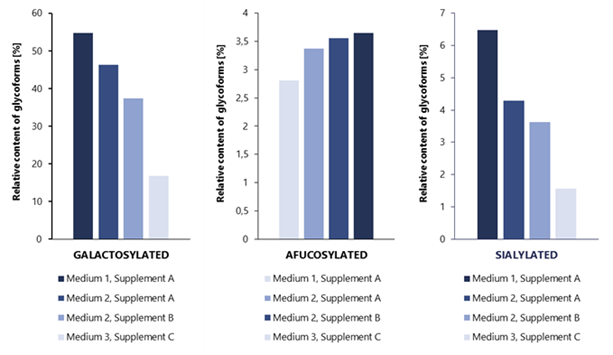
Figure 3 Impact of cell culture medium and supplementation on rituximab glycosylation profile.
Antibody glycosylation from regulatory standpoint – differences acceptable by authorities
Quality attributes of glycosylation profile, due to the strong impact on activity and safety, are carefully considered by regulatory authorities regarding the comparison of biosimilar candidate and originator drug. The requirement of glycosylation analysis and characterization is noted in EMA and FDA guidelines31,32. Based on analysis of multiple batches of reference, QTPP study provides the ranges for specific quality attributes including those related to glycosylation such as galactosylated, afucosylated, sialylated glycoforms content, site occupancy, etc. The established ranges serve as a specific benchmark for the manufacturer of biosimilar on the requirements that should be met to ensure safety and efficacy of the molecule. Nevertheless, it is not a prerequisite that given CQA of biosimilar drug must always be within the reference range values. Moreover, the extent of differences between biosimilar and reference that is tolerated by regulatory agencies is not strictly defined, but any result out of reference limits must be supported by adequate justification and sometimes additional studies demonstrating no effect on drug’s performance.
Similar conclusions emerge from screening study of EMA-approved rituximab biosimilars undertaken at Mabion compared with the data obtained for the reference drug. Glycosylation quality attributes of two biosimilars in comparison to the reference drug were characterized together with biological activity assessment. Within the scope of the study five (5) batches of Biosimilar 1 and four (4) batches of Biosimilar 2 were investigated. In case of both approved biosimilars, individual and grouped glycoform content was not fully consistent compared with the reference product batches analyzed by Mabion team (34 batches manufactured between 2011 and 2016) (see Figure 4). For example, for G0F and G1 glycoform content, three and all five tested batches of Biosimilar 1 were out of reference product range (established by Mabion based on mean ±3SD of reference batches). Biosimilar 1 results were below limit of quantification of the method. Moreover, in case of Biosimilar 2, all batches were outside the reference product range determined in similar assessment for Man5 glycoform level. Yet, these differences did not lead to significant changes in biological activity of the drugs. All batches of both biosimilars remained within mean ±2SD range for ADCC activity along with ±3SD ranges for CDC and pro-apoptotic activity, as established by Mabion. Aforementioned ranges for biological activity attributes were determined based on 37, 25 and 29 reference batches, manufactured between 2011 and 2016, for CDC, ADCC and pro‑apoptotic effect, respectively.
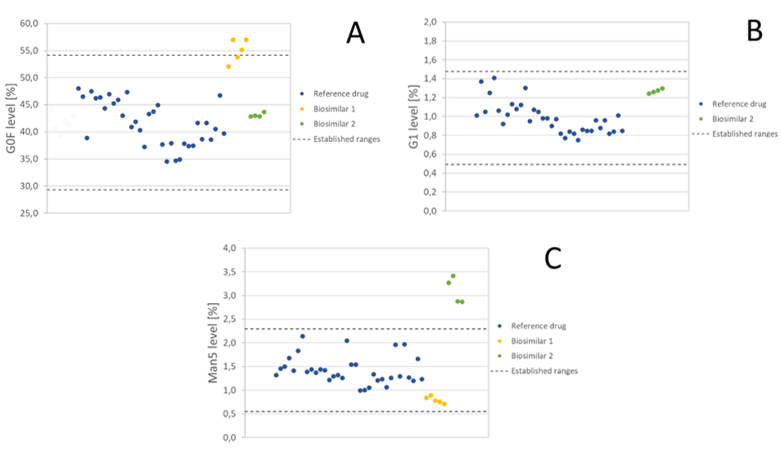
Figure 4 Selected results from the screening study of rituximab biosimilars performed at Mabion. (A) G0F level for biosimilar 1 and biosimilar 2 compared to established ranges (mean value for 34 batches of reference drug ±3SD). (B) G1 level for biosimilar 2 compared to established ranges (mean value for 34 batches of reference drug ±3SD). Biosimilar 1 results were below the LLOQ of the method. (C) Man5 level for biosimilar 1 and biosimilar 2 compared to established ranges (mean value for 34 batches of reference drug ±3SD).
Minor and inconsequential differences in glycosylation profile between biosimilar and reference product might be acceptable by regulatory bodies if appropriately justified, and do not preclude the demonstration of biosimilarity8. Several products in the history of biosimilars have shown dissimilarities in the content of one or more glycoforms, but they were nevertheless approved by EU and/or US agencies. One example is epoetin alfa HX575 manufactured by Janssen, which contained higher levels of phosphorylated high mannose type structures than the originator. The EMA considered this difference to be acceptable as similar glycoforms exists in multiple recombinant erythropoietins and cytokines, and they bear little relevance to the biological activity of the molecule. Small shifts in glycosylation pattern were also noted for EU-approved biosimilars to follitropin alfa: Ovaleap and Bemfola. The latter displayed an even higher number of dissimilarities than epoetin alfa (i.e., higher ratio of tetra-antennary:di-antennary structures, differences in distribution of fucosyl residues in relation to antennarity, O-acetyl–containing sialic residues of α-subunit below level of detection), but these were all labelled as minor and considered non-important from the medical point of view8. More recent example applicable to the monoclonal antibodies is rituximab biosimilar from Pfizer (Ruxience, PF-05280586)38. Several analytical differences were noted by the FDA during the regulatory review, concerning the level of G0 glycans, Man5 and terminal galactosylation, however they did not preclude the demonstration of similarity:
“G0 glycan (by HILIC, risk ranking 2): The applicant’s data showed that differences in the level of G0 glycans that are below 7.6% do not have significant impact on ADCC, which is the attribute most likely to be impacted by G0 differences). The applicant also provided data demonstrating that the observed difference (approximately 1.5%) in G0 between the products does not have an impact on ADCC activity. – Man5 (by HILIC, risk ranking 2): The actual values and the difference are small (0.4% vs 1.2% average content in PF-05280586 and in Rituxan lots, respectively), and such levels will not affect the antibody product clearance and PK (…). This assessment is also supported by the comparative PK study results reviewed by the clinical pharmacology team. – N-linked glycan profile (by HILIC, risk ranking 3): The visual similarity did not meet due to differences in G0 and Man5. – Terminal galactosylation (by HILIC, risk ranking 2): Eighty seven percent of PF-05280586 batches fall within the U.S.-licensed Rituxan quality range (just outside of 90% acceptance criterion). The worst-case PF-05280586 batch with 37% terminal galactosylation showed similar CDC activity (the biological activity impacted by terminal galactosylation) to the U.S.-licensed Rituxan lots and other PF-05280586 lots.”
Food and Drug Administration (FDA). APPLICATION NUMBER: 761103Orig1s000: MEDICAL and STATISTICAL REVIEW(S). May 10, 2019. 39
Specifically regarding the analytical differences they wrote:
“Analytical differences are noted, but they do not preclude demonstration of highly similar. The differences (…) would not impact the activity of the product and do not preclude a determination that PF-05280586 is highly similar to U.S.-licensed Rituxan.”
Food and Drug Administration (FDA). APPLICATION NUMBER: 761103Orig1s000: MEDICAL and STATISTICAL REVIEW(S). May 10, 2019. 39
The bottom line is that the biosimilar with a slightly dissimilar glycosylation profile may obtain approval, provided that the applicant presents incontrovertible evidence that the identified differences would have no expected impact on potency, efficacy or safety.
Analysis of glycosylation profile
Analytical methods for glycosylation profile assessment
Analysis of mAb’s glycosylation profile should follow a holistic approach, which includes testing for total glycan content as well as characterization of site-specific patterns and site occupancy. Early strategic discussions with regulatory bodies as well as sharing the biosimilar and reference data to consult the approach to similarity assessment is essential for a successful drug development.
The complexity of the glycan structures and their enormous impact on antibody drug action renders the glycosylation profile analysis one of the most intricate aspects of biopharmaceutical characterization. The methods of glycosylation analysis can be categorized into three groups depending on the molecule size of the analyte: intact and subunit level, glycopeptides and released glycans37.
Analysis at the intact protein level gives information on molecular weight and major post-translational modifications, such as glycosylation. Mass spectrometry (MS) methods are most suitable for this application and most commonly employed by the biopharmaceutical industry40. Antibody samples are initially separated using either chromatographic (LC) or electrophoretic (CE) method and then directed for MS detection. Additionally, subunit level approach based on reduction and enzymatic digestion (e.g. by IdeS) of mAb enables improved separation and sensitivity of analysis. Glycopeptide analysis approach provides the characteristics of glycosylation profile including site-specific patterns and site occupancy7. mAb is enzymatically cleaved into small fragments and analyzed by MS coupled with LC or CE separation method. Furthermore, released glycan level analysis evaluates relative glycan content and monosaccharide composition40. Glycans are released by PNGase F digestion and fluorescently labeled (due to lack of natural chromophore is carbohydrate structures) prior to analysis. Most commonly, released glycans are analyzed by LC or CE separation linked with either fluorescence or MS detection41.
LC-MS methods have been developed and now successfully utilized by Mabion for determining molecular mass and glycosylation for rituximab at both intact and subunit level. Reversed-phase chromatographic separation coupled with MS detection leads to identification of the main glycoforms located on the heavy chain of antibody, including G0F, G1F and G2F. Additional reduction of disulfide bonds prior to analysis provides better resolution and demonstrates levels of glycan pair asymmetry.
Glycopeptide analysis approach utilizing sample preparation and subsequent MS detection is effectively used at Mabion to determine the glycosylation site occupancy for comprehensive drug characterization studies. Occupancy of the asparagine glycosylation site on rituximab Fc region is evaluated by semi qualitative measurements and specified as a ratio of glycosylated antibody to the sum of its glycosylated and non glycosylated forms.
HILIC method with fluorescence (FLD) detection is considered to be the most suitable approach for released total glycan content routine analysis in light of its robustness, accuracy and reproducibility9. Such analytical technique is available at Mabion for drug substance and product release in the GMP conditions. It provides efficient separation, relative quantification and comparison of key glycoforms, e.g. afucosylated species, for reference and biosimilar drug (see Figure 5). Noteworthy, the method employs RapiFluor-MS rather than traditional 2-AB as labeling agent, enhancing fluorescence intensity signal and mass spectrometry ionization for potential application of MS detection. Moreover, Mabion offers other methods based on released glycan analysis (see Figure 6) for monosaccharide quantification and sialic acid content determination (with additional distinction of Neu5Ac and Neu5Gc glycans using RP-UHPLC with FLD detection).
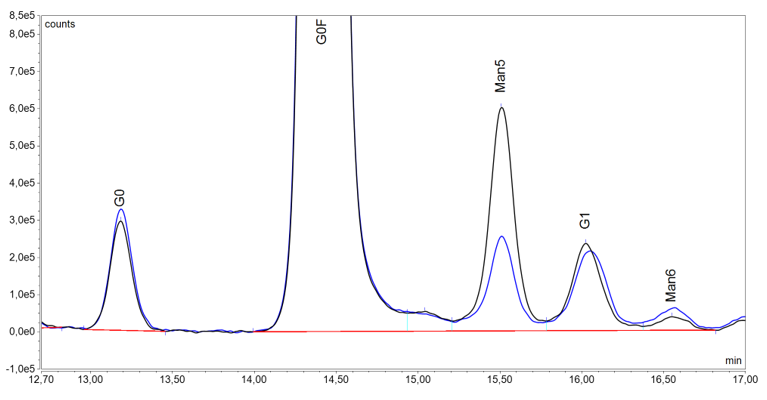
Figure 5 Comparison of glycosylation profile by HILIC-UHPLC-FLD for a reference (black line) and biosimilar (blue line) drug. Chromatogram fragments, where the differences in the afucosylated glycoforms (G0, Man5, G1, Man6) are particularly prominent, are presented.
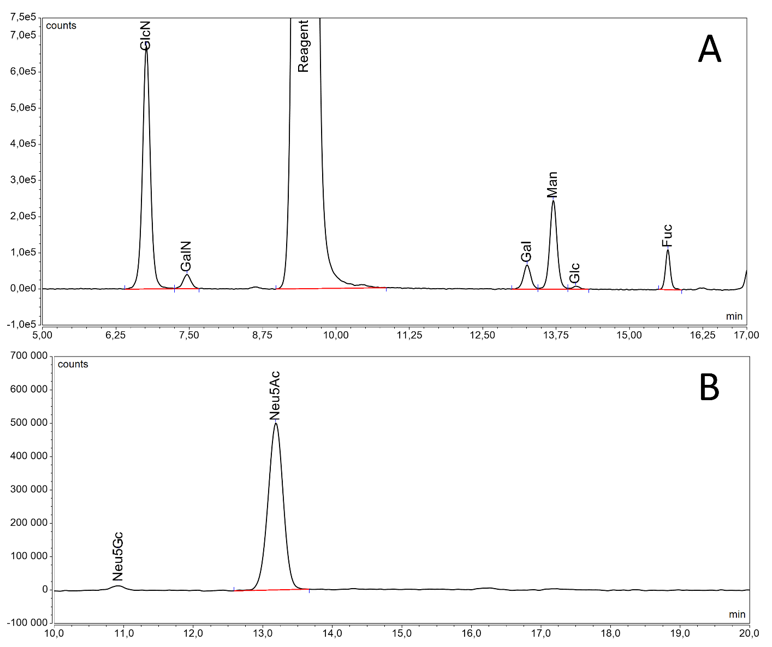
Figure 6 Chromatograms obtained for reference drug from released glycan based methods: monosaccharide quantification by RP-UHPLC-FLD (A) and sialic acid content determination by RP-UHPLC-FLD (B).
Caveats of glycoform analysis
It is worth emphasizing that none of the available analytical methods can be viewed as gold standard for the characterization of glycosylation profile of therapeutic antibodies. Every single approach outlined above has advantages but also limitations. Intact protein level analyses provide information such as molecular weight and PTMs (e.g. glycosylation) having the benefit of simple sample preparation. However, the identification of small differences in PTMs and low-abundant glycoforms is problematic with these methods, thus compromising the precise glycoform characterization. Glycopeptide-based techniques are well-suited tool for site-specific glycosylation studies and enhance the reliability of glycoform identification. Nevertheless, introducing and validating MS-based techniques in GMP laboratories could be a challenging endeavor. In contrast, methods based on released glycan analysis are relatively amenable to implement for release testing purposes. On the other hand, this approach requires additional sample preparation and allows only for the determination of content of predefined glycans. For this reason, in order to address all above issues, it is essential to be equipped with a wide portfolio of methods dedicated to the analysis of glycosylation.
Summary
It is worth emphasizing that none of the available analytical methods can be viewed as gold standard for the characterization of glycosylation profile of therapeutic antibodies. Every single approach outlined above has advantages but also limitations. Intact protein level analyses provide information such as molecular weight and PTMs (e.g. glycosylation) having the benefit of simple sample preparation. However, the identification of small differences in PTMs and low-abundant glycoforms is problematic with these methods, thus compromising the precise glycoform characterization. Glycopeptide-based techniques are well-suited tool for site-specific glycosylation studies and enhance the reliability of glycoform identification. Nevertheless, introducing and validating MS-based techniques in GMP laboratories could be a challenging endeavor. In contrast, methods based on released glycan analysis are relatively amenable to implement for release testing purposes. On the other hand, this approach requires additional sample preparation and allows only for the determination of content of predefined glycans. For this reason, in order to address all above issues, it is essential to be equipped with a wide portfolio of methods dedicated to the analysis of glycosylation.
Prepared by:
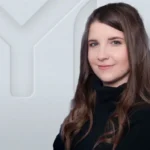
Paulina Toboła
Analytics Development Division Manager
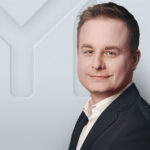
Adam Tuszyner
Registration Dossier Specialist, Pharmacovigilance Specialist
Piotr Miłek
Literature
1. Wang Z, Zhu J, Lu H. Antibody glycosylation: impact on antibody drug characteristics and quality control. Appl Microbiol Biotechnol. 2020 Mar;104(5):1905-14. doi: 10.1007/s00253-020-10368-7.
2. Kaur H. Characterization of glycosylation in monoclonal antibodies and its importance in therapeutic antibody development. Crit Rev Biotechnol. 2021 Mar;41(2):300-315. doi: 10.1080/07388551.2020.1869684.
3. Crommelin D., Sindelar R., Meibohm B. Pharmaceutical Biotechnology. 2019, CRC Press, ISBN 9780429145810.
4. Welply JK. Protein glycosylation: function and factors that regulate oligosaccharide structure. Biotechnology. 1991;17:59-72. doi: 10.1016/b978-0-409-90123-8.50009-4.
5. Pezer M, Stambuk J, Perica M, Razdorov G, Banic I, Vuckovic F, Gospic AM, Ugrina I, Vecenaj A, Bakovic MP, Lokas SB, Zivkovic J, Plavec D, Devereux G, Turkalj M, Lauc G. Effects of allergic diseases and age on the composition of serum IgG glycome in children. Sci Rep. 2016 Sep 12;6:33198. doi: 10.1038/srep33198.
6. Rathore A., et al. Glycosylation in mAb therapeutic products: analytical characterization and impact of process. LCGC Europe. 2016;29.7: 385-392.
7. Duivelshof BL, Jiskoot W, Beck A, Veuthey JL, Guillarme D, D’Atri V. Glycosylation of biosimilars: Recent advances in analytical characterization and clinical implications. Anal Chim Acta. 2019 Dec 16;1089:1-18. doi: 10.1016/j.aca.2019.08.044.
8. Grampp G, Ramanan S. The Diversity of Biosimilar Design and Development: Implications for Policies and Stakeholders. BioDrugs. 2015 Dec;29(6):365-72. doi: 10.1007/s40259-015-0147-0.
9. Liu L. Antibody glycosylation and its impact on the pharmacokinetics and pharmacodynamics of monoclonal antibodies and Fc-fusion proteins. J Pharm Sci. 2015 Jun;104(6):1866-1884. doi: 10.1002/jps.24444.
10. Brunton LL, Hilal-Dandan R, Knollmann BC. eds. Goodman & Gilman’s: The Pharmacological Basis of Therapeutics. 2017, McGraw Hill, ISBN 9781259584732.
11. Oliveira S., Santarino I., Oliveira‐Brett AM. Direct electrochemistry of native and denatured anticancer antibody rituximab at a glassy carbon electrode. Electroanalysis 2013;25.4: 1029-1034.
12. Wang H, Wu L, Wang C, Xu J, Yin H, Guo H, Zheng L, Shao H, Chen G. Biosimilar or Not: Physicochemical and Biological Characterization of MabThera and Its Two Biosimilar Candidates. ACS Pharmacol Transl Sci. 2021 Mar 12;4(2):790-801. doi: 10.1021/acsptsci.0c00225.
13. Data from MabionCD20 similarity studies.
14. Boyd PN, Lines AC, Patel AK. The effect of the removal of sialic acid, galactose and total carbohydrate on the functional activity of Campath-1H. Mol Immunol. 1995 Dec;32(17-18):1311-8.
15. Hodoniczky J, Zheng YZ, James DC. Control of recombinant monoclonal antibody effector functions by Fc N-glycan remodeling in vitro. Biotechnol Prog. 2005 Nov-Dec;21(6):1644-52. doi: 10.1021/bp050228w.
16. Raju TS. Terminal sugars of Fc glycans influence antibody effector functions of IgGs. Curr Opin Immunol. 2008 Aug;20(4):471-8. doi: 10.1016/j.coi.2008.06.007.
17. Kang J, Kim SY, Vallejo D, Hageman TS, White DR, Benet A, Coghlan J, Sen KI, Ford M, Saveliev S, Tolbert TJ, Weis DD, Schwendeman SP, Ruotolo BT, Schwendeman A. Multifaceted assessment of rituximab biosimilarity: The impact of glycan microheterogeneity on Fc function. Eur J Pharm Biopharm. 2020 Jan;146:111-124. doi: 10.1016/j.ejpb.2019.12.003.
18. Flynn GC, Chen X, Liu YD, Shah B, Zhang Z. Naturally occurring glycan forms of human immunoglobulins G1 and G2. Mol Immunol. 2010 Jul;47(11-12):2074-82. doi: 10.1016/j.molimm.2010.04.006.
19. Wright A, Sato Y, Okada T, Chang K, Endo T, Morrison S. In vivo trafficking and catabolism of IgG1 antibodies with Fc associated carbohydrates of differing structure. Glycobiology. 2000 Dec;10(12):1347-55. doi: 10.1093/glycob/10.12.1347.
20. Shields RL, Lai J, Keck R, O’Connell LY, Hong K, Meng YG, Weikert SH, Presta LG. Lack of fucose on human IgG1 N-linked oligosaccharide improves binding to human Fcgamma RIII and antibody-dependent cellular toxicity. J Biol Chem. 2002 Jul 26;277(30):26733-40. doi: 10.1074/jbc.M202069200.
21. Scallon BJ, Tam SH, McCarthy SG, Cai AN, Raju TS. Higher levels of sialylated Fc glycans in immunoglobulin G molecules can adversely impact functionality. Mol Immunol. 2007 Mar;44(7):1524-34. doi: 10.1016/j.molimm.2006.09.005.
22. Kaneko Y, Nimmerjahn F, Ravetch JV. Anti-inflammatory activity of immunoglobulin G resulting from Fc sialylation. Science. 2006 Aug 4;313(5787):670-3. doi: 10.1126/science.1129594.
23. Naso MF, Tam SH, Scallon BJ, Raju TS, 2010, Engineering host cell lines to reduce terminal sialylation of secreted antibodies. Mabs 2(5):519–27.
24. Yehuda S, Padler-Karavani V, 2020, Glycosylated Biotherapeutics: Immunological Effects of N-Glycolylneuraminic Acid, Front Immunol. 11:21.
25. Xu Y, Xie L, Zhang E, Gao W, Wang L, Cao Y, Xie MH, Jiang W, Liu S. Physicochemical and functional assessments demonstrating analytical similarity between rituximab biosimilar HLX01 and the MabThera®. MAbs. 2019 Apr;11(3):606-620. doi: 10.1080/19420862.2019.1578147.
26. Krapp S, Mimura Y, Jefferis R, Huber R, Sondermann P, 2003, Structural analysis of human IgG-Fc glycoforms reveals a correlation between glycosylation and structural integrity. J Mol Biol. 325(5):979–89.
27. Abès R, Teillaud JL. Impact of Glycosylation on Effector Functions of Therapeutic IgG. Pharmaceuticals (Basel). 2010 Jan 12;3(1):146-157. doi: 10.3390/ph3010146.
28. Ha S, Ou Y, Vlasak J, Li Y, Wang S, Vo K, Du Y, Mach A, Fang Y, Zhang N, 2011, Isolation and characterization of IgG1 with asymmetrical Fc glycosylation. Glycobiology. 21(8):1087-96.
29. Mimura Y, Church S, Ghirlando R, Ashton PR, Dong S, Goodall M, Lund J, Jefferis R. The influence of glycosylation on the thermal stability and effector function expression of human IgG1-Fc: properties of a series of truncated glycoforms. Mol Immunol. 2000 Aug-Sep;37(12-13):697-706.
30. Bartusik-Czubek E, Toboła P, Czubek B, Bednarek M, Balcerek J, Pietrucha T, Jaros S. Modeling of the Biological Activity of Monoclonal Antibodies Based on the Glycosylation Profile. J Pharm Sci. 2021 Apr;110(4):1661-1667. doi: 10.1016/j.xphs.2021.01.010.
31. Food and Drug Administration (FDA). “GUIDANCE DOCUMENT: Scientific Considerations in Demonstrating Biosimilarity to a Reference Product”. April 2015.
32. EMA. Guideline on similar biological medicinal products containing biotechnology-derived proteins as active substance: quality issues (revision 1) https://www.ema.europa.eu/en/documents/scientific-guideline/guideline-similar-biological-medicinal-products-containing-biotechnology-derived-proteins-active_en-0.pdf, 2014. (Accessed 12 October 2023).
33. Ehret J, Zimmermann M, Eichhorn T, Zimmer A. Impact of cell culture media additives on IgG glycosylation produced in Chinese hamster ovary cells. Biotechnol Bioeng. 2019 Apr;116(4):816-830. doi: 10.1002/bit.26904.
34. Edwards E, Livanos M, Krueger A, Dell A, Haslam SM, Mark Smales C, Bracewell DG. Strategies to control therapeutic antibody glycosylation during bioprocessing: Synthesis and separation. Biotechnol Bioeng. 2022 Jun;119(6):1343-1358. doi: 10.1002/bit.28066.
35. Batra J, Rathore AS. Glycosylation of monoclonal antibody products: Current status and future prospects. Biotechnol Prog. 2016 Sep;32(5):1091-1102. doi: 10.1002/btpr.2366.
36. van Berkel PH, Gerritsen J, Perdok G, Valbjørn J, Vink T, van de Winkel JG, Parren PW. N-linked glycosylation is an important parameter for optimal selection of cell lines producing biopharmaceutical human IgG. Biotechnol Prog. 2009 Jan-Feb;25(1):244-51. doi: 10.1002/btpr.92.
37. Peiqing Zhang, Susanto Woen, Tianhua Wang, Brian Liau, Sophie Zhao, Chen Chen, Yuansheng Yang, Zhiwei Song, Mark R. Wormald, Chuanfei Yu, Pauline M. Rudd. Challenges of glycosylation analysis and control: an integrated approach to producing optimal and consistent therapeutic drugs, Drug Discovery Today, Volume 21, Issue 5, 2016, Pages 740-765, ISSN 1359-6446, https://doi.org/10.1016/j.drudis.2016.01.006.
38. Ruxience Prescribing Information (2023).
39. Food and Drug Administration (FDA). APPLICATION NUMBER: 761103Orig1s000: MEDICAL and STATISTICAL REVIEW(S). May 10, 2019.
40. Beck A, Wagner-Rousset E, Ayoub D, Van Dorsselaer A, Sanglier-Cianférani S. Characterization of therapeutic antibodies and related products. Anal Chem. 2013 Jan 15;85(2):715-36. doi: 10.1021/ac3032355.
41. Reusch D, Haberger M, Falck D, Peter B, Maier B, Gassner J, Hook M, Wagner K, Bonnington L, Bulau P, Wuhrer M. Comparison of methods for the analysis of therapeutic immunoglobulin G Fc-glycosylation profiles-Part 2: Mass spectrometric methods. MAbs. 2015;7(4):732-42. doi: 10.1080/19420862.2015.1045173.
Related resources
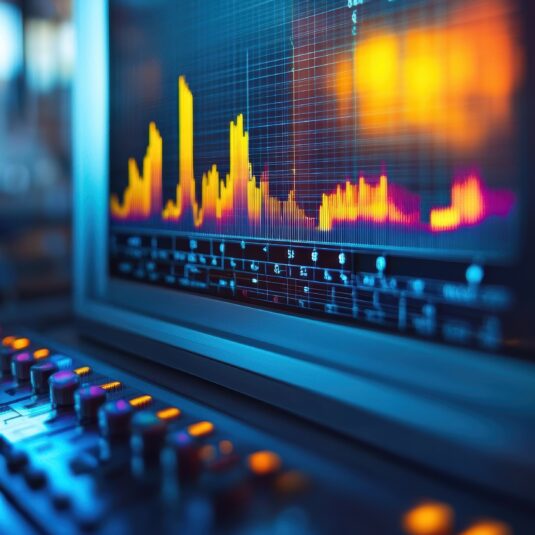
Mass spectrometry in peptide and protein analysis
Analytics, Drug substance, Proteins
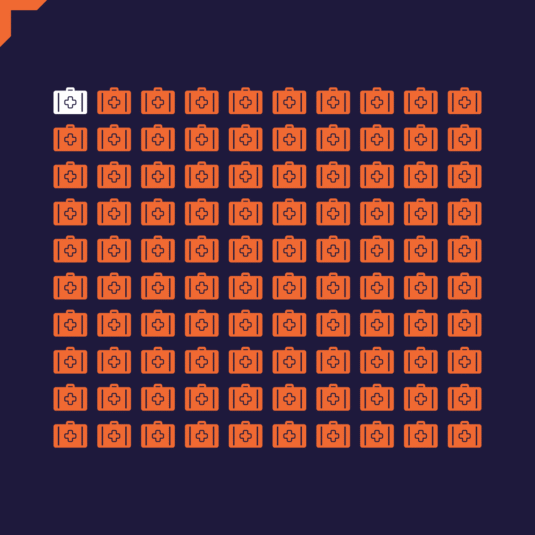
Time to Market Strategy – 5 CDMO Tactics to Speed Up Biologic Launches
Biologics, Drug development, Manufacturing
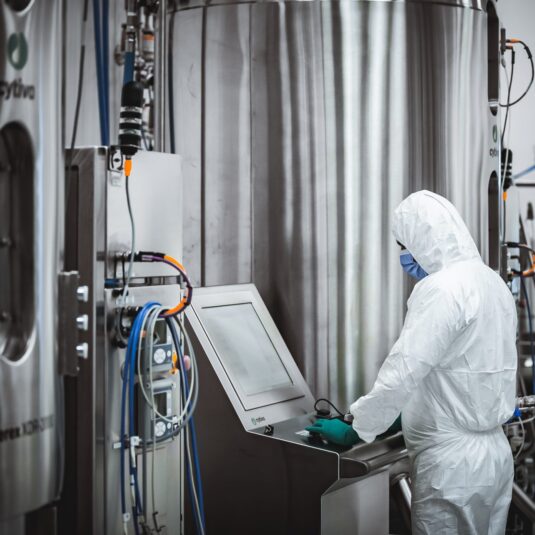
Navigating OOX Results: Effective Analysis and Management in CDMO Laboratories
Analytics, Manufacturing, Regulatory